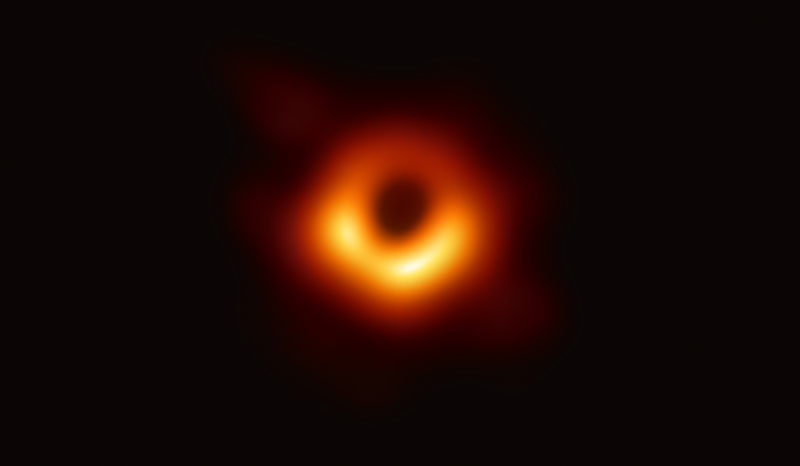
Here Is All You Wanted to Know About the First Ever Image of a Black Hole
- News
- 1.8K
The Event Horizon Telescope has released the first direct image of a black hole and its neighbourhood. This black hole lurks in the centre of a nearby galaxy called M87. This historic image shows a ring of light coming from the gas falling into the black hole.
The black hole itself is shielded by the event horizon, a boundary from within which nothing can escape, and this is the dark interior in the image. Though astronomers have had solid evidence for the existence of massive black holes for many years, this historic moment marks the first time that it has been imaged.
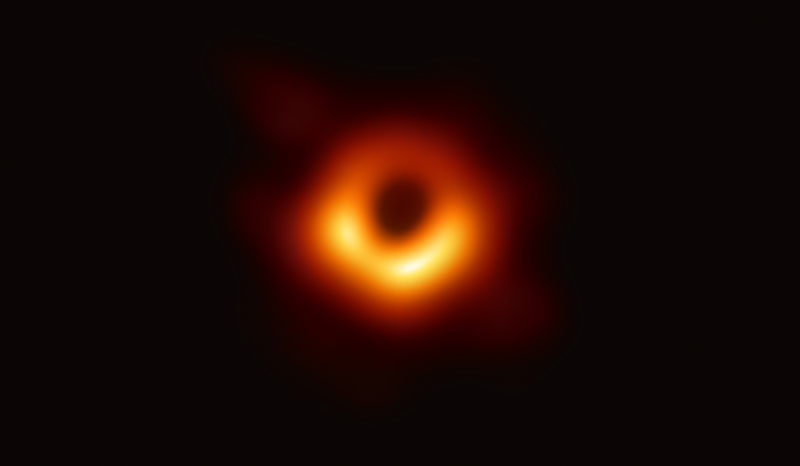
What has the Event Horizon Telescope seen?
The Event Horizon Telescope, or EHT, has imaged the silhouette or shadow of the black hole at the centre of M87, a galaxy 55 million light years from us. To make this image, astronomers combined data from 8 different telescopes across the world in an experiment in April 2017. The data was taken at a frequency of 230 GHz, or a wavelength of 1.3 mm. Using this, astronomers have formed an image of the black hole for the first time.
The event horizon of a black hole is the ultimate boundary. Nothing from within it can escape out. The ring of fire in the EHT image is light from the gas falling into the event horizon, whose shadow is the dark hole in the centre. The exact shape of the ring is due to the way the incredible gravity of the black hole bends the light around it, and the incredible speed at which the gas is travelling is why the ring is not uniform in brightness.
How big is the black hole at the centre of M87?
Almost all galaxies have black holes at their centres, and these can be a few million to a few billion times the mass of our Sun. Our Milky Way galaxy has a fairly small black hole about 4 million times as massive as our Sun. However, the black hole in M87 is a monster and is 6500 million times the mass of the Sun. The size of its event horizon is about 20000 million km, which even bigger than our Solar System.
A black hole does not emit any light. Then how do astronomers ‘see’ it or its shadow?
The matter is attracted by the gravity of a black hole, but cannot fall into it easily. In fact, it forms a swirling disk around it, through which it spirals into the black hole at extremely high speed. While doing so, the matter gets heated to enormous temperatures, and this hot magnetised plasma is what emits the intense radiation that we see.
EHT has got an image of the radiation from this surrounding gas at a frequency of 230 GHz. However, the image is not as simple as a dark hole in front of a disk of radiating gas. Since the gravity near the black hole is immense, it can bend the path of the light rays from the surrounding magnetised plasma in peculiar ways. So even light from the gas behind the black hole bends enough to reach us. This bending of the light, called gravitational lensing, determines the final shape of the ring and the inner shadow that the EHT has imaged.
Why are black holes and their images so important?
Black holes can test physics theories such as the General Relativity theory of Einstein, which relates the motion of bodies due to gravity with the curvature of spacetime. It has passed every test in our Solar System (the accuracy of GPS in our phones is also a good test of the theory) as well as in other astronomical objects.
All this is called the weak gravity case where the curvature of spacetime is small. What astronomers want to do is to test the theory in strong gravity, where the curvature is much higher, and see if the theory still works. The recent detection of gravitational waves from merging black holes is one such example. Imaging the shadow of super-massive black holes in galactic centres is another.
Why was it such a difficult experiment?
The EHT had to image M87 by collecting the radiation over a range of frequencies, and it did so by observing at a frequency of 230 GHz, which corresponds to a wavelength of 1.3 mm. This frequency is more than 2000 times higher than what is used by FM radio stations. This is a pretty special frequency where many factors align favourably.
At much lower frequencies, the inner region of the centre of M87 becomes more opaque and less bright. At higher frequencies, our own atmosphere blocks much of the radiation from coming in. 230 GHz seems just right. Telescopes at this frequency require incredibly hi-tech hardware, functioning at their limits of performance, including high precision atomic clocks and a digital backend.
Is there a single Event Horizon Telescope?
A telescope large enough to image the shadow of the black hole in M87 would have to be as big as the Earth itself. Since that might be a bit difficult, astronomers chose the next best thing. Using a technique called interferometry, data from many telescopes spread across the Earth were combined in a special way. This enabled astronomers to make images that show detail on as fine a scale as would a single Earth-sized telescope. However, this comes with the cost of enormous computation that requires months of processing on very powerful computers.
Why did the EHT not image the black hole in our own Galaxy?
The black hole in the centre of our Milky Way is about 1000 times less massive than the one in M87, and hence also smaller by the same factor. However, it is only 26000 light years away from us and hence appears to be a bit bigger on the sky than the black hole in M87. EHT had also observed the Milky Way black hole, but since its brightness varies much more rapidly, even during the observation duration, the data processing to make the image is more difficult.
Why did the telescopes have to be so far apart?
The EHT experiment had eight telescopes operating together at sub-mm wavelengths. These were as far apart as Hawaii, mainland USA, Chile, Mexico and even the South Pole. All of them had to look at M87 together at the same time and record their data. The telescopes functioning together in this way are jointly called the Event Horizon Telescope.
The size of the ring seen by EHT is around 40 micro-arcsecond – which is the angle made by the thickness of a sheet of paper viewed edge-on from around 100 km away. The black hole in M87 is the largest in the local Universe and is, therefore, a good bet.
To image such a small region, we need a telescope that has an enormous magnification, so that details within the image can be captured well. In the technique used to combine data from different telescopes, the magnification is higher if the telescopes are farther apart. The farthest apart they can be, of course, is the size of the Earth itself. The magnification of the EHT image is enough for you to sit in New Delhi and read a book which is in Kanyakumari.
Why Indian telescopes were not part of EHT?
India does not have a telescope working in the sub-mm wavelengths. Though India has two of the world’s largest radio telescopes (Giant Metrewave Radio Telescope near Pune and Ooty Radio Telescope), they operate at centimetre and metre wavelengths and will be completely blind at the shorter wavelengths of sub-mm. (India Science Wire)
[Niruj Mohan Ramanujam is an astronomer and a member of the Public Outreach and Education Committee of the Astronomical Society of India]
If you liked this article, then please subscribe to our YouTube Channel for the latest Science & Tech news. You can also find us on Twitter & Facebook.